Learning Objectives
- Define and recognize a population as a group of interacting individuals who are members of the same species.
- Know that four factors matter for the growth of a population of members of the same species: births and immigration increase population size, while deaths and emigration decrease population size.
- Compare and distinguish between exponential and logistic population growth equations and interpret the resulting growth curves.
- Analyze graphs to determine if regulation is influenced by density.
- Define life history traits and identify patterns of life history traits that correspond to exponential versus logistic population growth patterns.
Population ecology
A population is a group of interacting organisms of the same species. Most populations have a mix of young and old individuals. Quantifying the numbers of individuals of each age or stage gives the demographic structure of the population. In addition to demographic structure, populations vary in total number of individuals, called population size, and how densely packed together those individuals are, called population density. A population’s geographic range has limits, or bounds, established by the physical limits that the species can tolerate, such as temperature or aridity, and by the encroachment of other species. Population ecologists often first consider the dynamics of population size change over time, of whether the population is growing in size, shrinking, or remaining static over time.
Basic Dynamics – Exponential Population Growth
The most basic approach to population growth is to begin with the assumption that every individual produces two offspring in its lifetime, then dies, which would double the population size each generation. This population doubling at each generation is how an ideal bacterium with unlimited resources would reproduce.
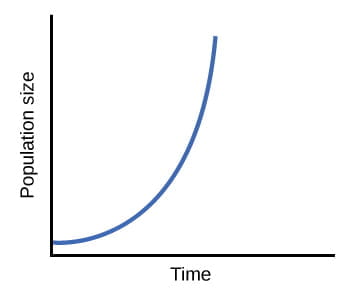
When resources are unlimited, populations exhibit exponential growth, resulting in a J-shaped curve. Source: OpenStax Biology
Because the births and deaths at each time point do not change over time, the growth rate of the population in this image is constant. Although the growth rate is fixed with time, the population doesn’t grow linearly in this model because every individual that was born in that generation reproduces. As a result, the population explodes in size very quickly—exponentially in fact. In nature, a population growing at this dramatic rate would quickly consume all available habitat and resources. In actuality, natural populations have size limits created by the environment.
Logistic Population Growth levels off at a carrying capacity
To consider how resource limitation affects population growth, we need to incorporate the concept of carrying capacity, the maximum population size that the environment can sustain. Any individuals born into this population would increase the population size unless the number of deaths balanced or outnumbered births. If the population size remains the same from one generation to the next, then individuals must also be dying at a similar rate. With exponential population growth, the population growth rate was constant. With the addition of a carrying capacity imposed by the environment, the logistic population growth rate slows as the population size increases, and growth is zero when the population reaches carrying capacity.
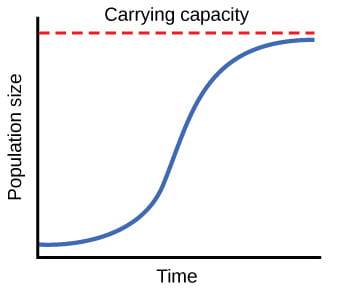
When resources are limited, populations exhibit logistic growth. In logistic growth, population expansion decreases as resources become scarce, and it levels off when the carrying capacity of the environment is reached, resulting in an S-shaped curve. Source: OpenStax Biology
Population size is regulated by factors that are dependent or independent of population density
Biological and non-biological factors can influence population size. Biological factors include interspecific interactions like predation, competition, parasitism, and mutualism, as well as disease. Non-biological factors are environmental variables like temperature, precipitation, disturbance, pollution, salinity, and pH. All of these factors can change population size, but only the biological factors (except mutualism) can “regulate” a population, meaning they push the population to an equilibrium density, or carrying capacity. Of the biological factors, mutualism does not regulate population size because mutualisms promote population increase through beneficial interactions with another species.
The biological factors of competition, predation, etc. that regulate population growth affect dense versus sparse populations differently. For instance, communicable disease does not spread quickly in a sparsely packed population, but in a dense population, like humans living in a college residence hall, disease can spread quickly through contact between individuals. Density plays a key role in population regulation in the following ways:
- Territoriality: Maintaining a territory will enable an individual to capture enough food to reproduce, where space is a limiting resource.
- Disease: Transmission rate often depends on population density
- Predation: Predators may concentrate on the most abundant prey
- Toxic Wastes: Metabolic by-products accumulate as populations grow
Identifying evidence of density regulation requires a field or lab experiment that manipulates density and quantifies the response in population growth. Often an (easier to measure) proxy of population growth, like survival or reproductive output, stands in as a quick metric of the births and deaths that will impact population growth. The characteristic negative correlation in the image below is evidence of density-dependent population regulation: higher densities yield lower survival.
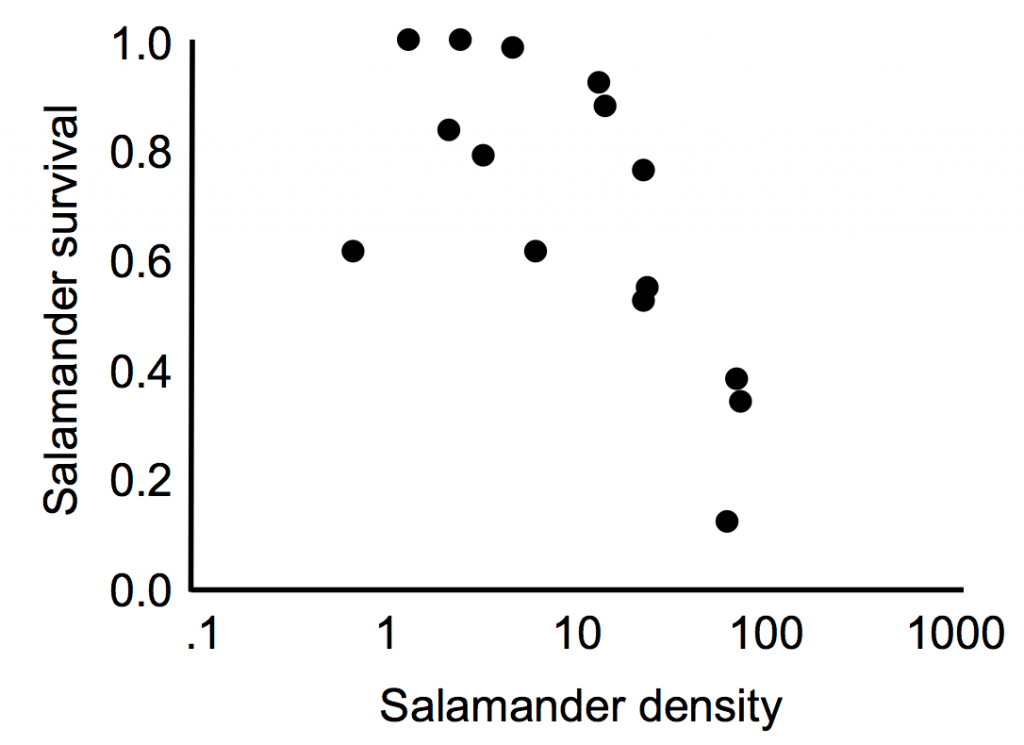
Van Burkirk and Smith (1991) manipulated salamander (Ambystoma laterale) larval density in the field and found lower salamander survival in the high density treatments on North Government Island, Isle Royale, Michigan. (Image after van Burkirk and Smith. 1991. Ecology 72(5): 1747-1756.)
Here’s Hank Green’s take on Population Growth to help you review these ideas:
Life history traits and their evolution
Individuals in a population experience a life cycle of birth, growth and development, maturity to adulthood, and then decline into reproductive senescence. How energy is allocated to these different aspects of the organisms survival is called their life history, and that energy allocation generates characteristic life history traits, traits that impact survival and reproductive output: size at birth, age at maturity, size at maturity, number and size of offspring (fecundity), reproductive value, lifespan and senescence, which we will define as the decline in fecundity with age. Life History Theory explains how evolution optimizes these survival and reproductive characteristics in different populations, affecting parameters such as: how big and fast individuals grow, when they reach sexual maturity, how many offspring they have each time they reproduce, how many times they reproduce, and when they die.
Notice that survival and reproduction are “optimized,” not maximized. This is because when evolution increases one of these traits, say survival of the parent, the result is usually a decrease in some aspect of reproduction, such as number of offspring produced each year, and vice versa. This optimization generates a life-history trade-off, depicted as a negative relationship between survival and reproduction (see figure below).
A life-history trade-off, depicted as a negative relationship between survival and reproduction, is probably caused by energy allocation split between survival and reproductive output for an organism.
The leading hypothesis for trade-offs in survival and reproduction is that energy is the limiting factor: organisms have finite energy, so if they allocate energy toward survival, then they don’t have as much available to reproduce. As a result, some organisms like the Chinook salmon reproduce only once in their short lifetime, while others such as Atlantic Cod—and humans—reproduce many times.
Selection for life history trade-offs between reproduction and survival can be related to a population’s growth rate and the environment’s carrying capacity.
- Populations that grow exponentially tend to have life history traits that maximize growth rate (live fast, die young). These populations include traits like having very large numbers of offspring, relatively small body size, early sexual maturity, broad dispersal of offspring, limited parental care, and short life-span. Species include insects, bacteria, many types of plants, and some small mammals such as rodents. These species tend to be found in places that are not limited by carrying capacity. While they tend to produce many, many offspring, each individual offspring has a low chance of survival. Their population sizes can change dramatically over short periods of time.
- Life history traits that are selected for in organisms living at densities near the environmental carrying capacity have traits opposing traits: relatively small numbers of offspring, comparatively large body size, late sexual maturity, offspring which require a high degree of parental care, and longer life-span. These species include large organisms such as many large mammals, some species of reptiles, and some species of birds. In the absence of natural disaster, disease epidemic, or other catastrophic event, the population size of these species tend to remain relatively stable and close to the maximum carrying capacity.
Literature Cited
Van Burkirk and Smith. 1991. Ecology 72(5): 1747-1756.