Learning Objectives
- Differentiate between sexual and asexual reproduction in plants
- Explain alternation of generations in ferns and contrast that to the life cycle of angiosperms (flowering plants).
- Describe the structure and function of the flower and the fruit within the angiosperm life cycle.
- Predict mechanisms of pollination or dispersal based on flower or fruit characteristics.
- Know that plants photosynthesize, building plant matter from CO2 + energy from the sun, and explain how that is different from how heterotrophic consumers acquire energy.
- Describe how plants achieve the circulation of water, minerals, and sugar throughout its body form.
[Adapted from Openstax Biology 32.2]
Many plants don’t need sex! They are able to propagate themselves using asexual reproduction. Asexual reproduction for plants requires less energy and resource investment than flower production, attracting pollinators, and finding a means of seed dispersal. Asexual reproduction produces plants that are genetically identical “clones” to each other and to the parent plant. In stable environmental conditions, asexual reproduction is a competitive reproductive strategy to sexual reproduction because asexual reproduction doesn’t involve recombination that would ‘scramble’ the well-adapted parental genotypes.
Some plants reproduce from the roots, like garlic, a scaly bulb in lilies, and a tunicate bulb in daffodils. Potato, parsnip, and ginger are other edible examples, and the strawberry plant produces a root runner to propagate asexually.

Some plants can be propagated through cuttings alone. An advantage of asexual reproduction is that the resulting plant will reach maturity faster. Since the new plant is arising from an adult plant or plant parts, it may also be sturdier than a sexually produced seedling. However, there are disadvantages to clonality, such as lack of variation in the face of changing environmental conditions, like the presence of a new disease.
Plants reproduce sexually through alternation of generations
[Adapted from OpenStax Biology 32.1]
Plants are so different from animals. They have two distinct multicellular stages in their life cycles, a phenomenon called alternation of generations. These two stages are the multicellular, haploid gametophyte and the multicellular diploid sporophyte. This is very different from most types of animal reproduction where there is only one multicellular stage, a diploid organism which produces single-celled haploid gametes.
In alternation of generations, the haploid gametophyte produces the male and female gametes by mitosis. The male and female gametes fuse to form the diploid zygote, which develops into the multicellular sporophyte. After reaching maturity, the diploid sporophyte produces spores by meiosis. Those spores divide by mitosis to produce the haploid, multicellular gametophyte. The new gametophyte produces gametes, and the cycle continues.
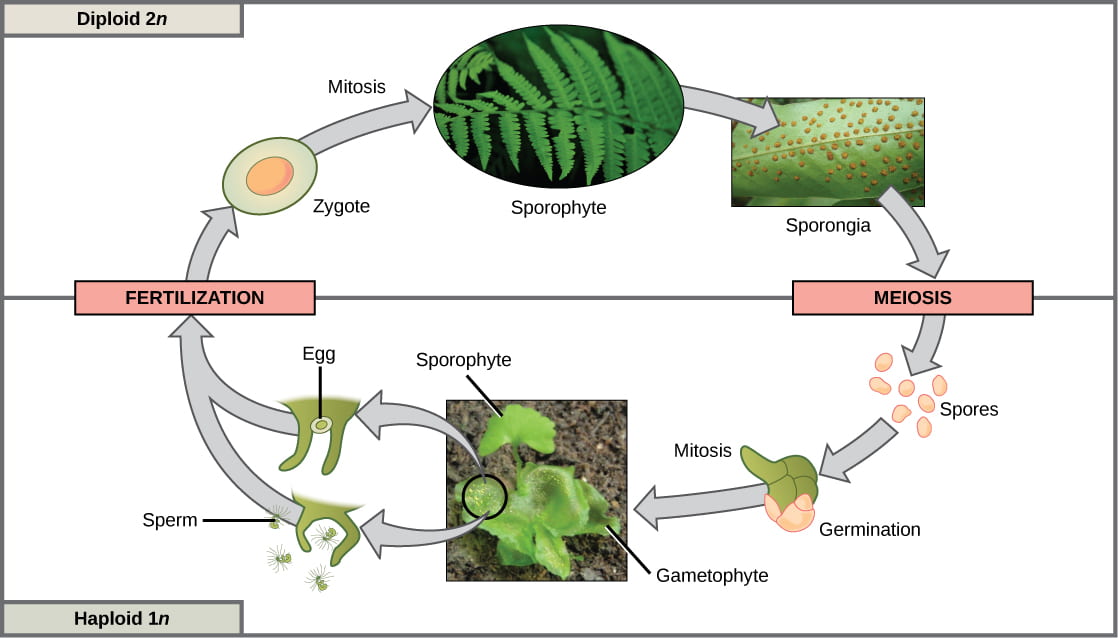
Plants have a life cycle that alternates between a multicellular haploid organism and a multicellular diploid organism. (Image credit “fern”: modification of work by Cory Zanker; credit “sporangia”: modification of work by “Obsidian Soul”/Wikimedia Commons; credit “gametophyte and sporophyte”: modification of work by “Vlmastra”/Wikimedia Commons)
The life cycles of angiosperms (flowering plants) and gymnosperms (conifers) are dominated by the sporophyte stage, and the gametophyte grows within or carried by the sporophyte. In ferns, the gametophyte is free-living and very distinct in structure from the diploid sporophyte. In mosses, the haploid gametophyte is the dominant and larger life form than the sporophyte.
The video below describes alternation of generation reproduction in gametophyte-dominant plants like mosses and ferns (you are not responsible for new vocabulary introduced in the video):
This next video describes reproduction in sporophyte-dominant plants like angiosperms and gymnosperms:
Reproduction in angiosperms
[Adapted from OpenStax Biology 32.1]
The lifecycle of angiosperms follows the alternation of generations life cycle above. The haploid gametophyte alternates with the diploid sporophyte during the sexual reproduction process of angiosperms. Flowers contain the plant’s reproductive structures.
Flower Structure
A typical flower has four main parts, or whorls—the sepals, petals, male reproductive organs, and female reproductive organs (see figure).
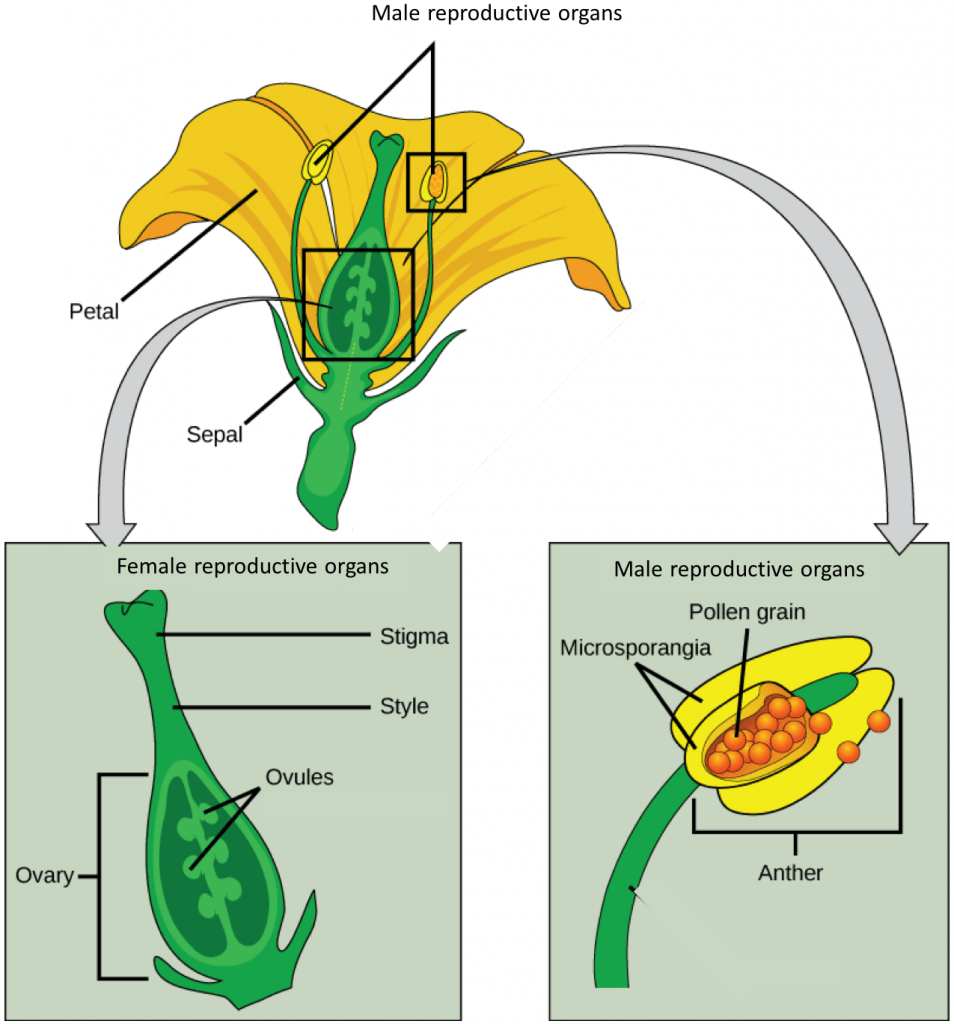
The four main parts of the flower are the sepals, petals, and male and female reproductive organs. (Image credit: modification of work by Mariana Ruiz Villareal)
The outermost whorl of the flower has green, leafy structures known as sepals, which help to protect the unopened bud. The second whorl is comprised of petals—this is the usually, brightly colored part we think of as the “flower.” The third whorl contains the male reproductive structures, which include stamens with anthers that contain the sperm-bearing pollen. The innermost group of structures in the flower is the female reproductive structure, made up of one or more carpels. Each carpel has a stigma, style, and ovary containing the eggs. Not all flowers contain both male and female organs.
Fertilization occurs when a pollen grain reaches the egg in the ovary
In a plant’s male reproductive organs, pollen develops inside the anther (see Figure above). When the flower is mature, the anther bursts open, releasing the pollen grains from the anther. Each pollen grain has a resilient covering that allows the pollen to survive under unfavorable conditions and move on wind, water, or with biological agents. When mature pollen grains reach the stigma, they tunnel down toward the ovary, delivering two sperm cells to fertilize an egg and secondary nutritive cell inside the ovary.
Pollination Syndromes
Pollination is transfer of pollen from the anther to the stigma: on the same plant this is called self-pollination, and it is called outcrossing when it transfer to another plant. Self-pollination has the advantage of convenience, and doesn’t involve investment in nectar and pollen as food for pollinators. The disadvantage is of course that self-pollinators do not gain genetic diversity through outcrossing, so they lose the advantage of sex as a way to obtain new genetic material. Recall that genetic diversity is necessary for evolution by natural selection to occur.
Plants have evolved many adaptations to avoid self-pollination. In some species, the pollen and the ovary mature at different times. Some flowers have evolved physical features that prevent self-pollination, such as two flower types with differences in anther and stigma length. Many plants, such as cucumber, have male and female flowers located on different parts of the plant, thus making self-pollination difficult. In a few plant species, the male and female flowers are actually on different plants!
The most reliable mechanism for outcrossing is genetic incompatibility to prevent pollen from germinating or growing into the stigma. If plants do not have compatible genes, the pollen is rejected by stigma cells, and the pollen tube cannot form.
Because plants cannot move to find mates, plants that do not self-pollinate depend on pollinators to help them mate. The majority of pollinators are biotic agents such as insects (like bees, flies, and butterflies), bats, birds, and other animals. Other plant species are pollinated by abiotic agents, such as wind and water.
Pollination by Insects
Bees are perhaps the most important pollinator of many native species, garden plants and most commercial fruit trees (Figure). Because bees cannot see the color red, bee-pollinated flowers usually have shades of blue, yellow, or other colors. Bees mutualistically collect energy-rich pollen or nectar for their survival and energy needs. Bee-pollinated flowers are open during the day, brightly colored, strongly scented, and tubular in shape. The pollen sticks to the bees’ fuzzy hair as it harvests nectar or pollen, and when the bee visits another flower, some of the pollen is transferred to the second flower. Note that the honeycomb picture in the rotating banner images on this website represents a diversity of bee pollen in the different cell colors in the comb (HHMI Biointerative Image of the Week).

Recently, there have been many reports about the declining population of honeybees. Many flowers will remain unpollinated and not bear seed if honeybees disappear. The impact on commercial fruit growers could be devastating.
Flies are an important pollinator in natural systems. Flies are attracted to flowers that have a decaying smell or an odor of rotting flesh, like the corpse flower or voodoo lily (Amorphophallus), dragon arum (Dracunculus), and carrion flower (Stapleia, Rafflesia). These flowers, which produce nectar, usually have dull colors, such as brown or purple. The nectar provides energy, whereas the pollen provides protein. Wasps are also important insect pollinators, and pollinate many species of figs.
Butterflies, such as the monarch, pollinate many garden flowers and wildflowers, which usually occur in clusters. These flowers are brightly colored, have a strong fragrance, and are open during the day. The pollen is picked up and carried on the butterfly’s limbs. Moths, on the other hand, pollinate flowers during the late afternoon and night. The flowers pollinated by moths are pale or white and are flat, enabling the moths to land.

Pollination by bats and birds
In the tropics and deserts, bats are often the pollinators of nocturnal flowers such as agave, guava, and morning glory. The flowers are usually large and white or pale-colored; thus, they can be distinguished from the dark surroundings at night. The flowers have a strong, fruity, or musky fragrance and produce large amounts of nectar. They are naturally large and wide-mouthed to accommodate the head of the bat. As the bats seek the nectar, their faces and heads become covered with pollen, which is then transferred to the next flower.
Many species of small birds, such as the hummingbird (Figure) and sun birds, are pollinators for plants such as orchids and other wildflowers. Flowers visited by birds are usually sturdy and are oriented in such a way as to allow the birds to stay near the flower without getting their wings entangled in the nearby flowers. The flower typically has a curved, tubular shape, which allows access for the bird’s beak. Brightly colored, odorless flowers that are open during the day are pollinated by birds. As a bird seeks energy-rich nectar, pollen is deposited on the bird’s head and neck and is then transferred to the next flower it visits. Botanists have been known to determine the range of extinct plants by collecting and identifying pollen from museum specimens of 200-year-old birds that were collected from the same site.

Abiotic pollination by wind and water
Anyone who has experienced spring in Georgia is familiar with wind pollination, which coats every surface daily for several weeks with plant pollen. Most species of conifers, and many angiosperms, such as grasses, maples and oaks, are pollinated by wind. Pine cones are brown and unscented, while the flowers of wind-pollinated angiosperm species are usually green, small, may have small or no petals, and produce large amounts of pollen. Unlike the typical insect-pollinated flowers, flowers adapted to pollination by wind do not produce nectar or scent. As the wind blows, the lightweight pollen is carried with it. The flowers usually emerge early in the spring, before the leaves, so that the leaves do not block the movement of the wind. Wind pollinated species produce huge quantities of pollen to balance the long odds that wind will deposited the pollen on the exposed feathery stigma.
Fruit development and seed dispersal
[Adapted from Openstax Biology 32.2]
After fertilization, the ovary of the flower usually develops into the fruit. Yep, when you eat an apple or a pear, you are eating the tree’s ovary. Fruits are usually associated with having a sweet taste; however, not all fruits are sweet. The fruit encloses the seeds and the developing embryo, thereby providing it with protection. Fruits come in many varieties: the sweet tissue of the blackberry, the red flesh of the tomato, the shell of the peanut, and the hull of corn (the tough, thin part that gets stuck in your teeth when you eat popcorn) are all fruits. As the fruit matures, the seeds also mature.
The fruit has a single purpose: seed dispersal. Seeds contained within fruits need to be dispersed away from the mother plant, so they may find favorable and less competitive conditions in which to germinate and grow.
Some fruit can disperse by themselves, whereas others require dispersal agents like wind, water, and animals (Figure). Modifications in seed structure, composition, and size help in dispersal. Wind-dispersed fruit are lightweight and may have wing-like appendages that allow them to be carried by the wind. Some have a parachute-like structure to keep them afloat. Some fruits—for example, the dandelion—have hairy, weightless structures that are suited to dispersal by wind. Seeds dispersed by water are contained in light and buoyant fruit, giving them the ability to float. Coconuts are well known for their ability to float on water to reach land where they can germinate. Similarly, willow and silver birches produce lightweight fruit that can float on water.



Animals and birds eat fruits as they forage, depositing the seeds that are not digested as excrement, often some distance away from the parent plant. Some animals, like squirrels, bury seed-containing fruits for later use; if the squirrel does not find its stash of fruit, and if conditions are favorable, the seeds germinate. Some fruits, like the cocklebur, have hooks or sticky structures that stick to an animal’s coat and are then transported to another place. Seed dormancy allows plants to disperse their progeny through time. Dormant seeds can wait months, years, or even decades for the proper conditions for germination and propagation of the species.
What is photosynthesis? [From the Khan Academy]
Have you hugged a tree lately? If not, you might want to give it some thought. You, along with the rest of the human population, owe your existence to plants and other organisms that capture light energy. In fact, most life on Earth is possible because the sun provides a continuous supply of energy to ecosystems.
All organisms, including humans, need energy to fuel the metabolic reactions of growth, development, and reproduction. But organisms can’t use light energy directly for their metabolic needs. Instead, it must first be converted into chemical energy through the process of photosynthesis.
Photosynthesis is the process in which light energy is converted to chemical energy in the form of sugars. In a process driven by light energy, glucose molecules (or other sugars) are constructed from water and carbon dioxide, and oxygen is released as a byproduct. The glucose molecules provide organisms with two crucial resources: energy and fixed—organic—carbon.
-
Energy. The glucose molecules serve as fuel for cells: their chemical energy can be harvested through processes like cellular respiration and fermentation, which generate adenosine triphosphate— , a small, energy-carrying molecule—for the cell’s immediate energy needs.
-
Fixed carbon. Carbon from carbon dioxide—inorganic carbon—can be incorporated into organic molecules; this process is called carbon fixation, and the carbon in organic molecules is also known as fixed carbon. The carbon that’s fixed and incorporated into sugars during photosynthesis can be used to build other types of organic molecules needed by cells.
1. Water diffuses into root cells. 2. Due to the properties of adhesion and cohesion, water molecules adhere to vessel walls and to one another. 3. As water evaporates through the open pores on leaf surfaces, each molecule exerts a pull on neighboring water molecules. Diagram by Laurel Jules, CC BY-SA 3.0 Wikimedia Commons.