Learning Objectives
- Know that genes are encoded in DNA, transcribed to RNA, and translated or expressed as protein phenotypes, and define this phenomenon as the “Central Dogma” of molecular biology.
- Describe the general roles of RNA polymerase, promoters, silencers, enhancers, ribosomes, and transfer RNAs in the process of gene expression.
- Know and give examples of how the regulation of gene expression can affect development and the organism’s final phenotype.
- Recognize that all multicellular life develops from a single cell, and know some common errors in development and their outcomes.
The Central Dogma states that DNA encodes genes that are transcribed into RNA and translated into proteins
The Central Dogma of molecular biology describes flow of information from DNA (genotype) to protein (phenotype). Information encoded in DNA is transcribed to RNA, and RNA is translated to a linear sequence of amino acids that fold into a functional protein. Proteins then do things in the cell, such as catalyze chemical reactions.
DNA → RNA → protein
This video gives an overview of the central dogma of molecular biology and where each step (transcription, translation) happens and each player (DNA, RNA, protein) resides inside a eukaryotic cell:
The next video shows transcription and translation, the process of gene expression in a eukaryotic cell, animated to help you understand that this is a dynamic process, with the key players:
Here are the key points of this process (from the audio transcript): When a gene is switched on (ready to be transcribed), an enzyme called RNA polymerase attaches to the gene. It moves along the chain of DNA, making a strand of messenger RNA (mRNA) out of free bases in the nucleus. The DNA code determines the order in which the free bases are added to the mRNA to make an RNA that corresponds precisely to the DNA. This process is called transcription.
Next, the mRNA moves out of the nucleus and into the cytoplasm, where translation occurs. Protein factories called ribosomes bind to the mRNA. The ribosome reads the code in the mRNA to produce a chain made up of amino acids in the specific order encoded by the mRNA. Transfer RNAs (tRNAs) carry the individual amino acids to the ribosome, where the mRNA is read three letters (bases) at a time, and as each is read, a tRNA delivers the corresponding amino acid. Once the last amino acid has been added, the chain folds into a 3-D shape to form the protein. Proteins can be structural, perhaps as part of a membrane, or functional, causing an effect in the cell. Some proteins are exported outside the cell to carry out tasks in other parts of the organism.
When a gene is expressed, and for how long, is controlled by signals within the body and within the cell. There are several ways to turn on a gene to make mRNA, as well as many ways to indicate how many copies of mRNA are made. All organisms have the same basic way to turn on a gene (purple line in the image below):
RNA polymerase (grey) binds to a site in the DNA called the promoter (blue line). Promoters have nearby regulatory elements (green line), binding sites for transcription factors which are DNA-binding proteins that can either help recruit, or repel, RNA polymerase. A regulatory element is a DNA sequence that certain transcription factors recognize and bind to in order to recruit or repel RNA polymerase. Activators help recruit RNA polymerase, and repressors help repel or block RNA polymerase. The promoter and nearby transcription factor binding elements work together to regulate gene transcription.
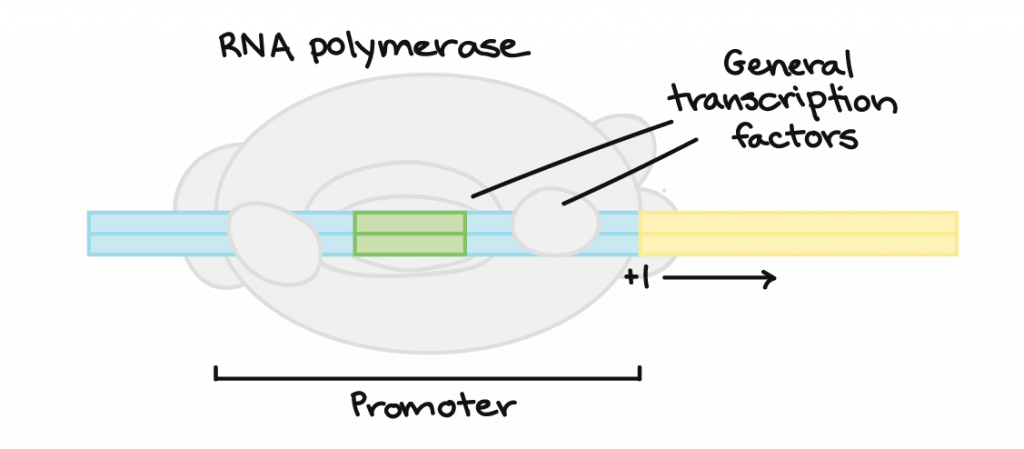
Image source: https://www.khanacademy.org/science/biology/gene-regulation/gene-regulation-in-eukaryotes/a/eukaryotic-transcription-factors
The timing of gene expression can modify development and the organism’s final phenotype
Cells express (transcribe and translate) only a subset of their genes. Cells respond and adapt to environmental signals by turning on or off expression of appropriate genes. In multicellular organisms, cells in different tissues and organs differentiate, or become specialized by making different sets of proteins, even though all cells in the body (with a couple of exceptions) have the same genome. Such changes in gene expression, or differential gene expression among cells, are most often regulated at the level of transcription.
Turning genes on in specific body parts
Sourced from Khan Academy. (See that source for references cited below)
Some genes need to be expressed in more than one body part or type of cell. For instance, suppose a gene needed to be turned on in your spine, skull, and fingertips, but not in the rest of your body. How can transcription factors make this pattern happen?
A gene with this type of pattern may have several enhancers (far-away clusters of binding sites for activators) or silencers (the same thing, but for repressors). Each enhancer or silencer may activate or repress the gene in a certain cell type or body part, binding transcription factors that are made in that part of the body. [1,2]
As an example, let’s consider a gene found in mice, called Tbx4. This gene is important for the development of many different parts of the mouse body, including the blood vessels and hind legs [3]. During development, several well-defined enhancers drive Tbx4 expression in different parts of the mouse embryo. The diagram below shows some of the Tbx4 enhancers, each labeled with the body part where it produces expression.
Enhancers like those of the Tbx4 gene are called tissue-specific enhancers: they control a gene’s expression in a certain part of the body. Mutations of tissue-specific enhancers and silencers may play a key role in the evolution of body form.[4]
How could that work? Suppose that a mutation, or change in DNA, happened in the coding sequence of the Tbx4 gene. The mutation would inactivate the gene everywhere in the body, and mouse without a normal copy would likely die. However, a mutation in an enhancer might just change the expression pattern a bit, leading to a new feature (e.g., a shorter leg) without killing the mouse.
So mouse development is modular! Genes are turned on for some amount of time, and that dictates the presence and size of a body part, like an arm or a leg. Drugs that interfere with development, like thalidomide, can cause birth defects.
Development is the process of creating complexity from a single cell
From Khan Academy. (See original article for citations)
During development, a human or other multicellular organism goes through an amazing transformation. Over the course of hours, days, or months, the organism turns from the single celled zygote (the fertilized egg) into a huge, organized collection of cells, tissues, and organs.
As an embryo develops, its cells divide, grow, and migrate in specific patterns to make a more and more elaborate body. To function correctly, that body needs well-defined axes (such as head vs. tail). It also needs a specific collection of many-celled organs and other structures, positioned in the right spots along the axes and connected up with one another in the right ways.
The cells of an organism’s body must also specialize into many functionally different types as development goes on. Your body (or even the body of a newborn) contains a wide array of different cell types, from neurons to liver cells to blood cells. Each one of these cell types is found only in certain parts of the body—in certain tissues of certain organs—where its function is needed.
Development is largely under the control of genes. Mature cell types of the body, like neurons and liver cells, express different sets of genes, which give them their unique properties and functions. In the same way, cells during development also express specific sets of genes. These patterns of gene expression cause cells to communicate with neighboring cells, resulting in coordinated development into a multicellular organism.
Some basic processes of development
Different organisms develop in different ways, but there are some basic things that must happen during the embryonic development of almost any organism:
-
The number of cells must increase through division
-
Body axes (head-tail, right-left, etc.) must form
-
Tissues must form, and organs and structures must take on their shapes
-
Individual cells must acquire their final cell type identities
To be clear, these processes are not separate events that happen one after another. Instead, they are going on at the same time as the embryo develops.
For instance, different body axes (such as head-tail and left-right) are set up at different times during early development, while the cells of the embryo are dividing away in the background. Similarly, formation of an organ requires cell division to build that organ, as well as differentiation (cells taking on their final identities) to ensure that the right cells make up the right parts of the organ.
When the developmental processes of body axes differentiation and cell type differentiation go astray, errors in development can occur. Some errors still lead to viable offspring, but other developmental errors are fatal at some point during development. Fatal developmental errors occur frequently, and are often the cause of spontaneous abortion of developing offspring. We’ll learn more about these in class.
The video below takes the ideas above and rounds them out with the bigger picture of animal development: